7 Understanding the Hominin Past: Climate, Dating, Diet, and DNA
Fossil evidence has demonstrated that a variety of hominins inhabited the planet beginning several million years ago. From their fossilized bones, we know much about how they moved and how hominins changed over time. To understand the fuller picture of hominins, paleoanthropologists investigate aspects of the environisotopesment and the genetic composition of the bones to better understand their adaptation and how they are related to us. This chapter focuses on these techniques in order to understand climate, dating, diet, and DNA.
Climate Change and Human Evolution
A key goal in the study of human origins is to learn about the environmental pressures that may have shaped human evolution. Scientists use a variety of techniques to reconstruct ancient environments. These include stable isotopes, core samples from oceans and lakes, windblown dust, analysis of geological formations and volcanoes, and fossils of ancient plant and animal communities. Such studies have provided valuable information about the environmental context of early Homo.
The early hominin species such as Ardipithecus ramidus and Australopithecus afarensis, evolved during the late Pliocene epoch. The Pliocene (5.3 million to 2.6 million years ago) was marked by cooler and drier conditions, with ice caps forming permanently at the poles. Still, Earth’s climate during the Pliocene was considerably warmer and wetter than at present.
The subsequent Pleistocene epoch (2.6 million years to 11,000 years ago) ushered in major environmental change. The Pleistocene is popularly referred to as the Ice Age. Since the term “Ice Age” tends to conjure up images of glaciers and woolly mammoths, one would naturally assume that this was a period of uniformly cold climate around the globe. But this is not actually the case. Instead, climate became much more variable, cycling abruptly between warm/wet (interglacial) and cold/dry (glacial) cycles. These patterns were influenced by changes in Earth’s elliptical orbit around the sun. As is shown in Figure 7.2, each cycle averaged about 41,000 years during the early Pleistocene; the cycles then lengthened to about 100,000 years starting around 1.25 million years ago. Since mountain ranges, wind patterns, ocean currents, and volcanic activity can all influence climate patterns, there were wide-ranging regional and local effects.
Figure 7.2: Temperature estimates during the last five million years, extrapolated from deep-sea core data. Lower temperatures and increased temperature oscillations start at 2.6 million years ago. Glacial/interglacial cycles during the early part of the epoch are shorter, each averaging about 41,000 years. Credit: Five Myr Climate Change by Dragons flight (Robert A. Rohde), based on data from Lisiechi and Raymo (2005), is under a CC BY-SA 3.0 License.
Data on ancient geography and climate help us understand how our ancestors moved and migrated to different parts of the world—as well as the constraints under which they operated. When periods of global cooling dominated, sea levels were lower as more water was captured as glacial ice. This exposed continental margins and opened pathways between land masses. During glacial periods, the large Indonesian islands of Sumatra, Java, and Borneo were connected to the Southeast Asian mainland, while New Guinea was part of the southern landmass of greater Australia. There was a land bridge connection between Britain and continental Europe, and an icy, treeless plain known as Beringia connected Northern Asia and Alaska. At the same time, glaciation made some northern areas inaccessible to human habitation. For example, there is evidence that hominin species were in Britain 950,000 years ago, but it does not appear that Britain was continuously occupied during this period. These early humans may have died out or been forced to abandon the region during glacial periods.
Exercises
In Africa, paleoclimate research has determined that grasslands (shown in Figure 7.2) expanded and shrank multiple times during this period, even as they expanded over the long term (deMenocal 2014). From studies of fossils, paleontologists have been able to reconstruct Pleistocene animal communities and to consider how they were affected by the changing climate. Among the African animal populations, the number of grazing animal species such as antelope increased. Although the African and Eurasian continents are connected by land, the Sahara desert and the mountainous topography of North Africa serve as natural barriers to crossing. But the fossil record shows that at different times animal species have moved back and forth between Africa and Eurasia. During the early Pleistocene, there is evidence of African mammal species such as baboons, hippos, antelope, and African buffalo migrating out of Africa into Eurasia during periods of aridity (Belmaker 2010).
Figure 7.2: A savanna grassland in East Africa. Habitats such as this were becoming increasingly common during the Pleistocene. Credit: Savanna grasslands of East Africa by International Livestock Research Institute (ILRI)/Elsworth is under a CC BY-NC-SA 2.0 License.
This changing environment was undoubtedly challenging for our ancestors, but it offered new opportunities to make a living. One solution adopted by some hominins was to specialize in feeding on the new types of plants growing in this landscape. The robust australopithecines likely developed their large molar teeth with thick enamel in order to exploit this particular dietary niche.
Members of the genus Homo took a different route. Faced with the unstable African climate and shifting landscape, they evolved bigger brains that enabled them to rely on cultural solutions such as crafting stone tools that opened up new foraging opportunities. This strategy of behavioral flexibility served them well during this unpredictable time and led to new innovations such as increased meat-eating, cooperative hunting, and the exploitation of new environments outside Africa.
Global Temperature Reconstruction
Oxygen isotopes are a powerful tool in tracking global temperature fluctuations throughout time. The isotopes of Oxygen 18 (18O) and Oxygen 16 (16O) occur naturally in Earth’s water. Both are stable isotopes, but 18O has a heavier atomic weight. In the normal water cycle, evaporation takes water molecules from the surface to the atmosphere. Because 16O is lighter, it is more likely to be part of this evaporation process. The moisture gathers in the atmosphere as clouds that eventually may produce rain or snow and release the water back to the surface of the planet. During cool periods like glacial periods (ice ages), the evaporated water often comes down to Earth’s surface as snow. The snow piles up in the winter but, because of the cooler summers, does not melt off. Instead, it gets compacted and layered year after year, eventually resulting in large glaciers or ice sheets covering parts of Earth. Since 16O, with the lighter atomic weight, is more likely to be absorbed in the evaporation process, it gets locked up in glacier formation. The waters left in oceans would have a higher ratio of 18O during these periods of cooler global temperatures (Potts 2012, 154–156; see Figure 7.3).
Figure 7.3: This graph depicts how temperatures of the sea have fluctuated greatly over the course of the history of the planet. Credit: Oxygen in deep sea sediment carbonate (Figure 2) by NASA Goddard Institute for Space Studies originally from “Science Briefs: Cold Climates, Warm Climates: How Can We Tell Past Temperatures?” by Gavin Schmidt, is in the public domain. [Image Description].
The microorganisms that live in the oceans, foraminifera, absorb the water from their environment and use the oxygen isotopes in their body structures. When these organisms die, they sink to the ocean floor, contributing to the layers of sediment. Scientists can extract these ocean cores and sample the remains of foraminifera for their 18O and 16O ratios. These ratios give us a good approximation of global temperatures deep into the past. Cooler temperatures indicate higher ratios of 18O (Potts 2012, 154–156).
Exercises
Chronometric Dating
Unlike relative dating methods, chronometric dating methods provide specific dates and time ranges. Many of the chronometric techniques we will discuss are based on work in other disciplines such as chemistry and physics. The modern developments in studying radioactive materials are accurate and precise in establishing dates for ancient sites and remains.
Many of the chronometric dating methods are based on the measurement of radioactive decay of particular elements. Elements are materials that cannot be broken down into more simple materials without losing their chemical identity (Brown et al. 2018, 48). Each element consists of an atom that has a specific number of protons (positively charged particles) and electrons (negatively charged particles) as well as varying numbers of neutrons (particles with no charge). The protons and neutrons are located in the densely compacted nucleus of the atom, but the majority of the volume of an atom is space outside the nucleus around which the electrons orbit (see Figure 7.4).
Figure 7.4: Simplified illustration of an atom. Credit: Atom Diagram by AG Caesar is under a CC BY-SA 4.0 License.
Elements are classified based on the number of protons in the nucleus. For example, carbon has six protons, giving it an atomic number 6. Uranium has 92 protons, which means that it has an atomic number 92. While the number of protons in the atom of an element do not vary, the number of neutrons may. Atoms of a given element that have different numbers of neutrons are known as isotopes.
Exercises
The majority of an atom’s mass is determined by the protons and neutrons, which have more than a thousand times the mass of an electron. Due to the different numbers of neutrons in the nucleus, isotopes vary by nuclear/atomic weight (Brown et al. 2018, 94). For instance, isotopes of carbon include carbon 12 (12C), carbon 13 (13C), and carbon 14 (14C). Carbon always has six protons, but 12C has six neutrons whereas 14C has eight neutrons. Because 14C has more neutrons, it has a greater mass than 12C (Brown et al. 2018, 95).
Most isotopes in nature are considered stable isotopes and will remain in their normal structure indefinitely. However, some isotopes are considered unstable isotopes (sometimes called radioisotopes) because they spontaneously release energy and particles, transforming into stable isotopes (Brown et al. 2018, 946; Flowers et al. 2018, section 21.1). The process of transforming the atom by spontaneously releasing energy is called radioactive decay. This change occurs at a predictable rate for nearly all radioisotopes of elements, allowing scientists to use unstable isotopes to measure time passage from a few hundred to a few billion years with a large degree of accuracy and precision.
Exercises
The leading chronometric method for archaeology is radiocarbon dating (Figure 7.23). This method is based on the decay of 14C, which is an unstable isotope of carbon. It is created when nitrogen 14 (14N) interacts with cosmic rays, which causes it to knock out a proton and convert to 14C. Carbon 14 in our atmosphere is absorbed by plants during photosynthesis, a process by which light energy is turned into chemical energy to sustain life in plants, algae, and some bacteria. Plants absorb carbon dioxide from the atmosphere and use the energy from light to convert it into sugar that fuels the plant (Campbell and Reece 2005, 181–200). Though 14C is an unstable isotope, plants can use it in the same way that they use the stable isotopes of carbon.
Figure 7.5: A graphic illustrating how 14C is created in the atmosphere, is absorbed by living organisms, and ends up in the archaeological record. Credit: Radiocarbon dating (Figure 7.32) original to Explorations: An Open Invitation to Biological Anthropology by Mary Nelson is under a CC BY-NC 4.0 License.
Animals get 14C by eating the plants. Humans take it in by eating plants and animals. After death, organisms stop taking in new carbon, and the unstable 14C will begin to decay. Carbon 14 has a half-life of 5,730 years (Hester et al. 1997, 324). That means that in 5,730 years, half the amount of 14C will convert back into 14N. Because the pattern of radioactive decay is so reliable, we can use 14C to accurately date sites up to 55,000 years old (Hajdas et al. 2021). However, 14C can only be used on the remains of biological organisms. This includes charcoal, shell, wood, plant material, and bone. This method involves destroying a small sample of the material. Earlier methods of radiocarbon dating required at least 1 gram of material, but with the introduction of accelerator mass spectrometry (AMS), sample sizes as small as 1 milligram can now be used (Hajdas et al. 2021). This significantly reduces the destructive nature of this method.
Exercises
The use of radiocarbon dating at Denisova Cave in modern-day Russia revealed an astounding find, the first dated first-generation individual with a Neanderthal mother and Denisovan father. Vivian Slon and colleagues (2018) sequenced the genome, which revealed the individual’s hybrid genetic background, and radiocarbon dated the remains, revealing the sub-adult was over 50,000 years old (Slon et al. 2018).
As mentioned before, 14C is unstable and ultimately decays back into 14N. This decay is happening at a constant rate (even now, inside your own body!). However, as long as an organism is alive and taking in food, 14C is being replenished in the body. As soon as an organism dies, it no longer takes in new 14C. We can then use the rate of decay to measure how long it has been since the organism died (Hester et al. 1997, 324). However, the amount of 14C in the atmosphere is not stable over time. It fluctuates based on changes to the earth’s magnetic field and solar activity. In order to turn 14C results into accurate calendar years, they must be calibrated using data from other sources. Annual tree rings (see discussion of dendrochronology below), foraminifera from stratified marine sediments, and microfossils from lake sediments can be used to chart the changes in 14C as “calibration curves.” The radiocarbon date obtained from the sample is compared to the established curve and then adjusted to reflect a more accurate calendar date (see Figure 7.6). The curves are updated over time with more data so that we can continue to refine radiocarbon dates (Törnqvist et al. 2016). The most recent calibration curves were released in 2020 and may change the dates for some existing sites by hundreds of years (Jones 2020).
Figure 7.6: This is a simplified example of a calibration curve, showing how the radiocarbon age (y axis) is compared with the calibration curve to produce calibrated dates (x axis). Credit: Radiocarbon Date Calibration Curve by HowardMorland is under a CC BY-SA 3.0 License. [Based on information from Reimer et al. 2004. Radiocarbon 46: 1029-58.] [Image Description].
And yet, 55,000 years is only a tiny fragment of human evolutionary history. It is insignificant in the context of the age of our planet. In order to date even older fossils, other methods are necessary.
Potassium-argon (K-Ar) dating and argon-argon (Ar-Ar) dating can reach further back into the past than radiocarbon dating. Used to date volcanic rock, these techniques are based on the decay of unstable potassium 40 (40K) into argon 40 (40Ar) gas, which gets trapped in the crystalline structures of volcanic material. It is a method of indirect dating. Instead of dating the fossil itself, K-Ar and Ar-Ar dates volcanic layers around the fossil. It will tell you when the volcanic eruption that deposited the layers occurred. This is where stratigraphy becomes important. The date of the surrounding layers can give you a minimum and maximum age of the fossil based on where it is in relation to those layers. This technique was used at Gesher Benot Ya’aqo in the Jordan Valley, dating early stratigraphic deposits of basalt flows to 100,000 years old (Bar-Yosef and Belmaker 2011). The site is unique because early layers of occupation with an Acheulean handaxe industry were made primarily of basalt, which is an uncommon material for this tool technology. The benefit of this dating technique is that 40K has a half-life of circa 1.3 billion years, so it can be used on sites as young as 100 kya and as old as the age of Earth. It is particularly useful in dating early hominin sites in Africa (Michels 1972, 120; Renfrew and Bahn 2016, 155). Another benefit to this technique is that it does not damage precious fossils because the samples are taken from the surrounding rock instead. However, this method is not without its flaws. A study by J. G. Funkhouser and colleagues (1966) and Raymond Bradley (2015) demonstrated that igneous rocks with fluid inclusions, such as those found in Hawai‘i, can release gasses including radiogenic argon when crushed, leading to incorrectly older dates. This is an example of why it is important to use multiple dating methods in research to detect anomalies.
Exercises
Diet Reconstruction
You may be familiar with the saying “you are what you eat.” When it comes to your teeth and bones, this adage is literal. Stable isotopes can also be used to reconstruct animal diet and migration patterns. Living organisms absorb elements from ingested plants and water. These elements are used in tissues like bones, teeth, skin, hair, and so on. By analyzing the stable isotopes in the bones and teeth of humans and other animals, we can identify the types of food they ate at different stages of their lives.
Plants take in carbon dioxide from the atmosphere during photosynthesis. We’ve already discussed this using the example of the unstable isotope 14C; however, this absorption also takes place with the stable isotopes of 12C and 13C. During photosynthesis, some plants incorporate carbon dioxide as a three-carbon molecule (C3 plants) and some as a four-carbon molecule (C4 plants). On the one hand, C3 plants include certain types of trees and shrubs that are found in relatively wet environments and have lower ratios of 13C compared to 12C. C4 plants, on the other hand, include plants from drier environments like savannahs and grasslands. C4 plants have higher ratios of 13C to 12C than C3 plants (Renfrew and Bahn 2016, 312). These ratios remain stable as you go up the food chain. Therefore, you can analyze the bones and teeth of an animal to identify the 13C/12C ratios and identify the types of plants that animal was eating.
Exercises
The ratios of stable nitrogen isotopes 15N and 14N can also give information about the diet of fossilized or deceased organisms. Though initially absorbed from water and soils by plants, the nitrogen ratios change depending on the primary diet of the organism. An animal who has a mostly vegetarian diet will have lower ratios of 15N to 14N, while those further up the food chain, like carnivores, will have higher ratios of 15N. Interestingly, breastfeeding infants have a higher nitrogen ratio than their mothers, because they are getting all of their nutrients through their mother’s milk. So nitrogen can be used to track life events like weaning (Jay et al. 2008, 2). A marine versus terrestrial diet will also affect the nitrogen signatures. Terrestrial diets have lower ratios of 15N than marine diets. In the course of human evolution, this type of analysis can help us identify important changes in human nutrition. It can help anthropologists figure out when meat became a primary part of the ancient human diet or when marine resources began to be used. The ratios of stable nitrogen isotopes can also be used to determine a change in status, as in the case of the Llullaillaco children (the “ice mummies”) found in the Andes Mountains. For instance, the nitrogen values in hair from the Llullaillaco Maiden showed a significant positive shift that is associated with increased meat consumption in the last 12 months of her life (Wilson et al. 2007). Although the two younger children had little changes in their diets in the last year of their short lives, the changes in their nitrogen values were significant enough to suggest that the improvement in their diets may have been attributed to the Incas’ desire to sacrifice healthy, high-status children” (Faux 2012, 6).
Exercises
Hunted Foods as an Important Resource
Hunter-gatherers known from the recent historical record clearly depend heavily on hunted foods—meat—for sustenance. Is this pattern a defining feature of hominins from the very beginning, or did it develop relatively recently, for example with the appearance of anatomically modern humans? This has been the subject of heated debate among archaeologists and paleoanthropologists. One reason the debate is so important is that hunting is seen as a key factor that makes us human.
No other primate relies significantly on hunting for subsistence. Chimpanzees, the ape most closely related to humans, hunt, kill, and eat small animals—red colobus monkeys are their preferred prey—but meat makes up at most 3% of their diet in terms of caloric intake. What chimps eat the most is ripe fruit—in some parts of their range, over 50% of chimps’ caloric intake comes from ripe figs. In contrast, among modern hunter-gatherers, hunted foods take up between 40% and 60% of the diet on average depending on the environment. In Arctic environments, meat and fish can take up nearly 100% of the total diet—there are literally no plant foods to collect and eat during most times of the year in the Arctic.
Based on stone tool cut marks on animal bone, Homo habilis appears to have been a scavenger of meat some 2 million years ago. The earliest evidence for direct hunting, as opposed to scavenging, comes from the site of Schöningen in Germany (Kouwenhoven 1997). Here there are 400,000-year-old remains of fore-hardened wooden spears alongside butchered horses. So, we know that the pattern of heavy reliance on hunting has deep roots going back hundred of thousands of years. The spears represent not only sophisticated projectile technology, but also very likely cooperation and coordination among a group of hominins for a common purpose.
In addition, hunting had affects on hominin biology, especially the brain. Meat, especially cooked meat, could have helped to fund the energy-expensive human brain. Big brains also come with a big price tag. Big brains require a surprising amount of energy, up to 20% of the human energy intake and more for developing infants. Meat fats provide a concentrated sources of calories, which can be used to fund the cost of brain growth. Gorillas, who are mainly vegans, cannot even get enough calories from their diet to grow a bigger brain. As anthropologist Greg Downey puts it, there just aren’t enough hours in the day for a gorilla to grow a bigger brain. Evidence for scavenging and hunting in the paleoanthropological record is of interest to paleontologists because it points toward the human pattern of increased energy input to offset brain growth. Another important source of energy for early hominins is fire.
Fire and Cooking Made Us Human
Today, we humans are totally reliant on fire and every culture cooks food. Fire is basically a chemical reaction between carbon (like charcoal) or carbon-containing materials (like wood or grass) and oxygen; the reaction releases heat and light that can be used by human in virtually every life-sustaining activity we engage in. Fire also releases carbon dioxide, a greenhouse gas. It can be thought as the first step in our ability to alter the environment on a global scale. The early discovery of the control and use of fire is probably at the top of anyone’s list of crucial developments that made us what we are. Fire was a new and powerful means of harnessing energy from the environment, and can be considered a kind of tool. Anthropologist James C. Scott (2017) puts it this way in his book Against the Grain; “In the lower Homo erectus levels, there is evidence that big cats were preying on hominins. In the upper levels where there is evidence for fire, the pattern gets reversed.” Fire, he argues, enabled hominins to become predator rather than prey. Scott thinks of fire as being domesticated, in the sense of being tamed.
Although the advantages of fire use seem obvious to us now, the question of what fire actually did for its earliest users—what were its specific adaptive advantages—is an interesting one. On the first pass, most people would point to warmth and protection from predators as likely benefits of fire for early humans, and there is little doubt that this was the case. Fire also would have allowed hunter-gatherers to intensify, get more food out of an area of land, by hunting with fire and increasing productivity of the landscape by burning. Fire might also have allowed humans to colonize new areas by using fire to make them more productive, as well as providing safety from new potential predators.
In his book Catching Fire (2009), Harvard anthropologist Richard Wrangham argues that the use of fire for cooking food in order to make it more digestible was a turning point in the development of the modern human brain, physique, and way of life. Fire, in a sense, is a way of “outsourcing” your teeth and gut, by doing the work of digestion literally outside your body. The chimpanzee gut by comparison is about three times the size of a human’s, and the chimp has to expend more energy digesting food. In effect, cooking allowed hominins to eat far less food and to eat a wider range of foods. Cooking food, Wrangham points out, increases the proportion of nutrients digested and reduces the energetic costs associated with digestion. This extra energy could be put toward a new “project”—building a larger brain. Other primates, like gorillas, simply can’t get enough energy from their environment to channel toward building a larger brain. There’s not enough hours in the day to eat enough leaves to support the body and have some leftover for funding the brain. Fire and cooking, Wrangham argues, allowed hominins to break through that energy barrier and fund the high cost of an expanding brain. Wrangham further points out that humans do not thrive on raw food diets, and non-essential functions like reproduction shut down on strict raw food diets.
Hominins had fire before totally modern humans came on the scene. The earliest evidence for the use of fire by hominins appears to be at Koobi Fora, a site in northern Kenya associated with Homo ergaster/erectus. Here, baked, reddened earth and charcoal particles are found next to stone tools lying on a buried surface dating to about 1.6 million years ago. Burnt bones of savanna grazing animals are found in association with Oldowan tools and fossils of Homo ergaster/erectus at a site in South Africa called Swartkrans Cave. Both of these contexts are considered to be fairly compelling evidence for the use of fire by Homo erectus, although there are still some debate over whether the fires could have been caused naturally. It can be difficult to distinguish intentional fires from natural ones, especially early on in the record (King 2017). Wrangham is cautious about the early dates for fire, although his hypothesis about the importance of cooking in human evolution would seem to require that the control of fire should appear at about the same time as hunting.
Aside from warmth, protection, a tool for getting food, and a way increase energy intake, fire likely brought people together. Hunter-gatherers often congregate around fires to eat together and tell stories. And fire often has a spiritual aspect. Among the Eveny reindeer herders of Siberia it is common practice to offer vodka to the fire when entering a new camp. This practice is called “feeding the fire.” As an older Eveny woman put it, “The fire is the foundation of life, we feed. We warm ourselves, we’re nourished with its help” (Vitebsky 2005:86).
Sweatiness and Its Consequences
Sophisticated tools, big brains, fire, and bipedalism are all fairly obvious features of humans that can be traced back in the paleoanthropological record. Somewhat less obvious is another feature of humans that stands out among primates and that is our “furlessness” and our “sweatiness.” Humans are the sweatiest of all the primates, which enables us to stay cool in very hot environments. Our sweat glands are also more watery, a better cooling system than that of other mammals. Most mammals cool themselves not by sweating but by panting, losing heat through their wet tongues and lungs. With our dense and watery sweat glands, humans can cool off more effectively and have greater endurance in mid-day heat than other mammals. Other mammals simply cannot do activities like hot yoga because of their lack of watery sweat. This ability especially critical because of our large brains. As skin cancer specialist Sharad Paul (2018) points out, just like big computers need big fans, larger brains need larger cooling systems. With enough persistence, a human hunter can literally run a grazing animal like a deer or antelope to death in mid-day heat, thanks to our sweatiness coupled with other biological features. But of course, most foragers rely on more ingenious methods like poisoned darts and arrows.
According the anthropologist Nina Jablonski (2010), our dense watery sweat glands was an essential adaptation to the more open savanna/woodland environment in Africa that Homo erectus occupied. Like modern humans, Homo erectus would have avoided confrontations with predators by hunting and scavenging during the heat of the day when lions and leopards are asleep under the trees. In order to effectively cool off, an increased ability to “sweat it out” was selected for.
The selection for sweat glands came with another related adaptation, our lack of fur. A thick coat of body hair or fur reduces the cooling effects of sweating, and thus to be effective, sweat glands do better on naked skin. Jablonski argues that furlessness is a response to the need for sweat glands, and the two work together to keep us cool. (Humans babies do have a covering of fur called lanugo in utero, but lose it shortly before before birth, and we are born naked and furless).
The final consequence of our watery sweat and furlessness is skin color. Chimpanzees, beneath their fur, have light skin, not needing further protection from the sun’s damaging UV rays. But if you are furless, protection is needed. Jablonski argues that the loss of fur precipitated another adaptation—the selection of darker skin to protect against damage to skin by ultraviolet light and loss of folate, which can lead to birth defects. In effect, the shift to a more open environment created a cascade of consequences, sweatiness, furlessness, and increased pigmentation.
Mitochondrial DNA
Anatomically Modern Humans (AMH’s) are morphologically distinct from previous Archaic Homo sapiens and Homo erectus forms. AMH’s average cranial capacity is slightly smaller, the brow ridge is reduced, and the faces are smaller. AMHs have a more gracile (less robust) cranial and postcranial skeleton. The modern human skull has a high vertical forehead and the face is situated below the frontal braincase (less prognathic). Anatomically modern humans likely arose from some form of Archaic human living in Africa, though we don’t know which one.
Anatomically modern humans (that is, people who look like us) likely arose in Africa between 200,000 and 300,000 years ago. This is indicated by the fossil evidence and genetic evidence. First, some of the earliest AMH fossils are found in Africa at the sites of Omo and Herto, and more recently at 300,000 years old the site of Jebel Irhoud in Morocco. Secondly, genetic anthropologists have looked at DNA from people around the world in order to investigate where early ancestors originated. But the geneticists did not focus on nuclear DNA, the DNA we usually talk about that we inherit from our mother and father. Rather, they looked at mitochondrial DNA. Mitochondrial DNA (mtDNA) resides in the mitochondria of cells. Mitochondria help cells to use oxygen and produce energy. Cells that require a lot of energy, like muscles cells, have many mitochondria. Because there are multiple mitochondria in each cell, there tends to be many more copies of mtDNA than nuclear DNA, making it ideal for studying ancient genetic variation.
Exercises
Unlike nuclear DNA with its 3 billion nucleotide bases, mitochondrial DNA has only 16,500 bases, so it is much smaller. Like bacterial DNA, mitochondria has a circular chromosome. Also, in contrast to nuclear DNA which is derived from the mother and father, mitochondrial DNA is only inherited through the mother. So everyone has their mother’s mitochondrial DNA whatever their genetic sex might be. Another difference is that mitochondrial DNA is not as good as nuclear DNA at checking for mistakes when cells divide. For this reason, mitochondrial has 20 times the mutation rate as nuclear DNA.
Because of these frequent mutations and simple inheritance, we can look at mitochondrial DNA and tease apart ancestry. If living people have identical mutations, then it is very likely they are related through their mothers. By the same logic, mitochondrial DNA can be used to connect living people to people from the distant past. Scientists also use what is called a molecular clock (Alex and Moorjani 2017). First, the number of mutations that arise in a single generation are calculated. Then, if you compare two people you can estimate the time elapsed since they shared a common ancestor by counting the differences.

One early concern in linking modern people to past people through mtDNA was that the mutation rate of mtDNA might be too fast and, therefore, too messy to be of any use. Surprisingly, it was shown through domesticated Syrian hamsters that the mutation rate was not too fast. It turns out that all domesticated Syrian hamsters are descended from a single female hamster collected from Aleppo in the 1930s. If the mutation rate was very fast, then it would be expected that the mtDNA of domesticated hamsters would vary a lot, and it would not be very useful for sorting out ancestry. After analyzing the mitochondrial DNA from feces from widely distributed domesticated Syrian hamsters (yes, they collected hamster feces through the mail), they found the DNA was identical! Since Syrian hamsters have been domesticated only since 1938, the researchers knew the mutation rate wasn’t too fast and could potentially be used to estimate ancestry.
One of the first applications of the idea of connecting modern people with people living in the past comes from the Romanovs, the Imperial Russian family. The Romanovs—the tsar, tsarina, and their five children— were murdered on July 16, 1918, by the Bolsheviks. Following the Revolution, the location of the remains of the Romanovs was a mystery. In 1991, in the Russian Ural Mountains, skeletons fitting the description of the Romanovs came to light. But was it them? Researchers tracked down the living descendants of the tsar and tsarina and compared the SNPs of their mtDNA to the tsar and tsarina SNPs. Both were matches. The fact that both the tsar and tsarina’s mtDNA matched a modern relative’s mtDNA, along with the other material evidence, meant this was a closed case; the mystery of the Romanovs had been solved through the help of mtDNA. (Incidentally, Tsarina Alexandra was the grandchild of Queen Victoria, who spread a SNP for haemophilia to the tsarina and her son Alexei). Mitochondrial DNA also helped solve the case of Richard III, who was buried in an unknown location. Richard had scoliosis, so when a body with scoliosis was found beneath a parking lot in Leicester, England in 2012, in a plausible location for Richard’s burial, the body’s mitochondrial DNA was compared to known a descendant of Richard. They were a perfect match (King et al. 2014).
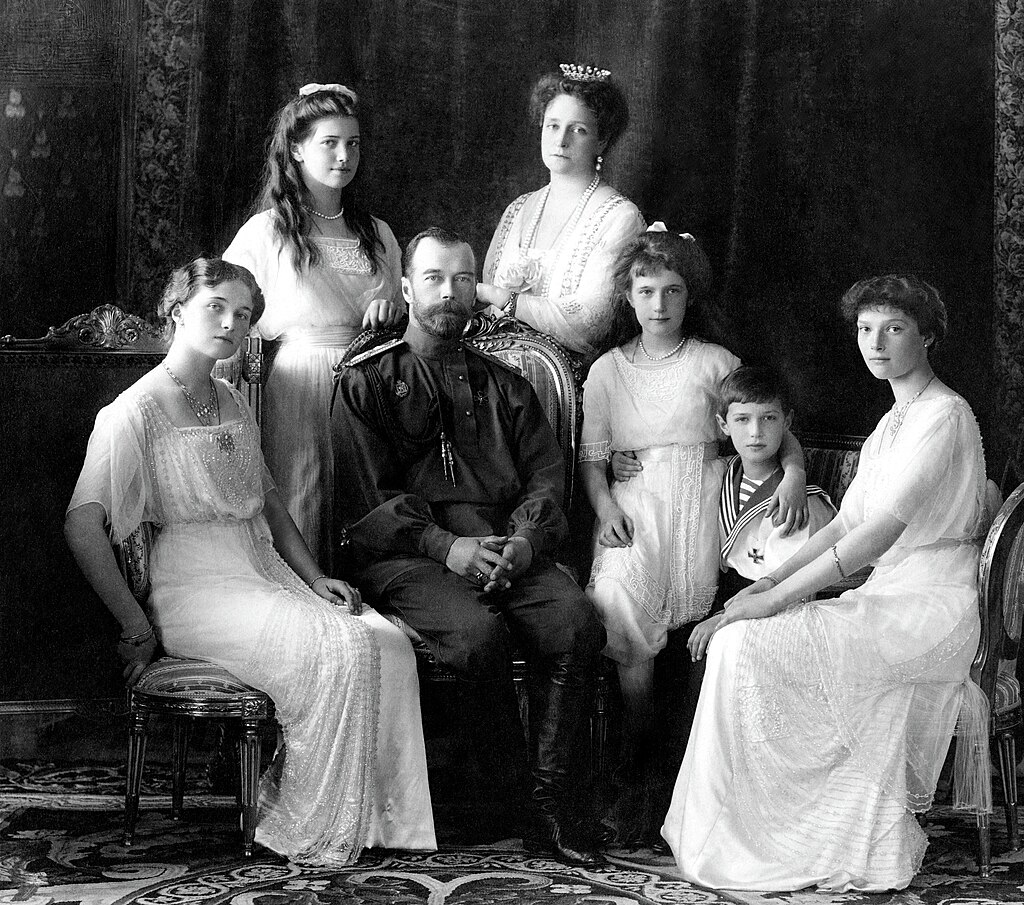
Special Topic: Cold Case Naia
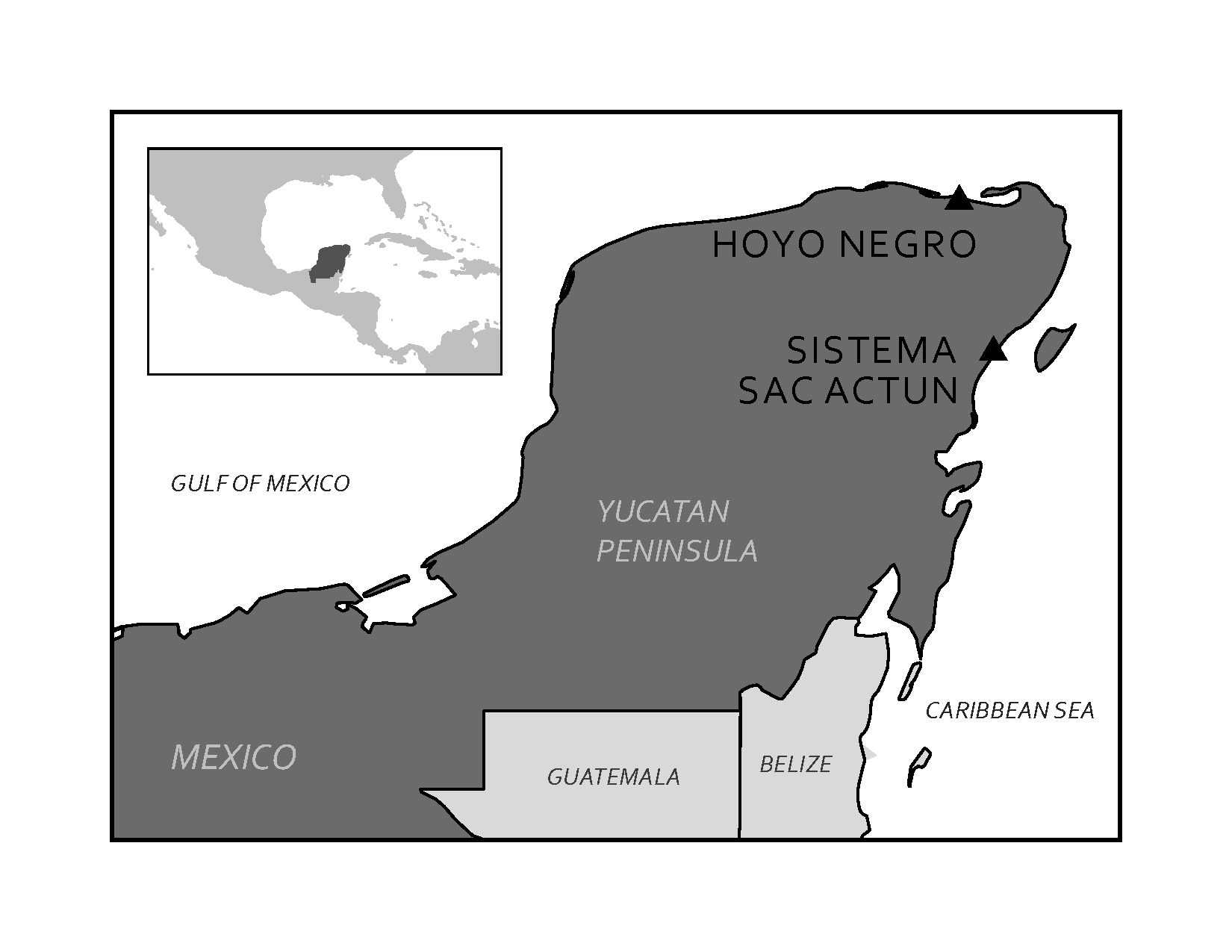
The following case is from Sarah S. King and Kara Jones in their chapter “Bones and Stone: Studying the Fossil Record.” In 2007, cave divers exploring the Sistema Sac Actun in the Yucatán Peninsula in Mexico (see Figure 7.28 and 7.29) discovered the bones of a 15- to 16-year-old female human along with the bones of various extinct animals from the Pleistocene (Collins et al. 2015). The site was named Hoyo Negro (“Black Hole”). The human bones belonged to a Paleo-American, later named “Naia” after a Greek water nymph. Examination of the partially fossilized remains revealed a great deal about Naia’s life, and the radiocarbon dating of her tooth enamel indicated that she lived some 13,000 years ago (Chatters et al. 2014). Naia’s arms were not overly developed, so her daily activities did not involve heavy carrying or grinding of grain or seeds. Her legs, however, were quite muscular, implying that Naia was used to walking long distances. Naia’s teeth and bones indicate habitually poor nutrition. There is evidence of violent injury during the course of Naia’s life from a healed spiral fracture of her left forearm. Naia also suffered from tooth decay and osteoporosis even though she appeared young and undersized. Dr. Jim Chatters hypothesizes that Naia entered the cave at a time when it was not flooded, probably looking for water. She may have become disoriented and fell off a high ledge to her death. The trauma to her pelvis is consistent with such an injury (Watson 2017).
Naia’s skeleton is remarkably complete given its age. As divers were able to locate her skull, Naia’s physical appearance in life could be interpreted. Surprisingly, in examining the skull, it was determined that Naia did not resemble modern Indigenous peoples in the region. However, the mitochondrial DNA (mtDNA) recovered from a tooth indicates that Naia shares her DNA with modern Indigenous peoples (Chatters et al. 2014). Though Naia’s burial environment made chemical analysis difficult, researchers were able to recover carbon isotopes from her remains. The isotopes from Naia’s tooth enamel suggest a diet of “cool-season grasses and/or broad-leaf vegetation” (Chatters et al. 2022, 68). Naia’s teeth also displayed numerous dental caries and only light dental wear. Coupled with the isotopic data, she likely had a “softer, more sugar-rich diet” (Chatters et al. 2022, 68).
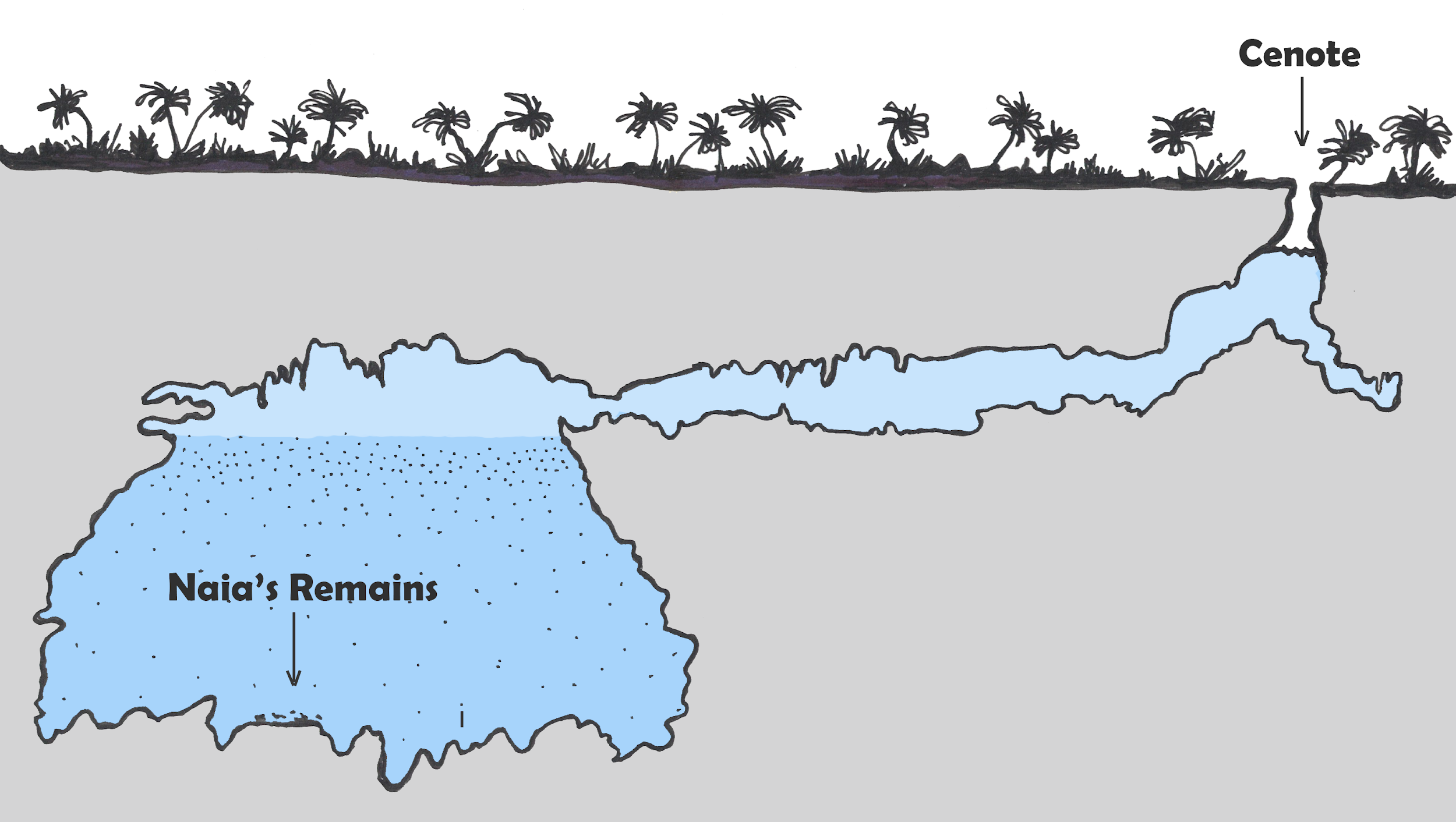
Out of Africa
Analysis of mtDNA can be used to investigate broader patterns of human migration. The region with the greatest mtDNA variation is likely the place where humans originated, based on the idea of increased time for new SNPs to develop in the mother population. In other regions, we should see SNPs that are derived from the original mother population, based on the Founder Effect. When mtDNA was analyzed from people around the world, the region that had the most variation was Africa. Biologist Lewis Spurgin (2013) explains why this is important:
“Travelling across unknown lands is a dangerous business, so we would expect ancient humans to avoid travelling a long way except when they needed to, and they probably didn’t travel en masse. Instead, when humans colonised new lands, it was probably a few intrepid explorers looking for new pastures. And the DNA evidence bears this out. Across the world, we see ‘bottlenecks’ at the genetic level — signatures where a small group of individuals, carrying a relatively small number of genetic variants, have set up new colonies. This is the key to understanding why the most genetically diverse human population can be found in Africa, while the populations of further migrations are descended from a much smaller stock of brave (or desperate) migrants.”
Both the fossil evidence and the mtDNA evidence point to Africa as the origin of modern humans. From there, humans spread to other parts of the world, encountering Neanderthals, Denisovans and likely other Archaic Humans. It is not known why no archaic humans are left to tell their side of the story. Did modern humans wage war with them? Did they out-compete them? We currently don’t know why we are the only representatives of the genus.
Attributions
This chapter is a remix of the following sources.
Sarah S. King and Kara Jones. “Chapter 7: Bones and Stone: Studying the Fossil Record,” In Explorations: An Open Introduction to Biological Anthropology, second edition, edited by Beth Shook, Lara Braff, Katie Nelson, and Kelsie Aguilera. The second edition chapter is a revision from “Chapter 7: Understanding the Fossil Context” by Sarah King and Lee Anne Zajicek. In Explorations: An Open Invitation to Biological Anthropology, first edition, edited by Beth Shook, Katie Nelson, Kelsie Aguilera, and Lara Braff, which is licensed under CC BY-NC 4.0.
Bonnie Yoshida-Levine. “Chapter 10: Early Members of the Genus Homo” In Explorations: An Open Invitation to Biological Anthropology, second edition, edited by Beth Shook, Lara Braff, Katie Nelson, and Kelsie Aguilera, which is licensed under CC BY-NC 4.0. The second edition chapter is a revision from “Chapter 10: Early Members of the Genus Homo” by Bonnie Yoshida-Levine. In Explorations: An Open Invitation to Biological Anthropology, first edition, edited by Beth Shook, Katie Nelson, Kelsie Aguilera, and Lara Braff, which is licensed under CC BY-NC 4.0.)
Sue Ruth. “Chapter 9: How Did We Get Here?” In Being Human.
Variants of elements.
Geological epoch dating from 5.3 to 2.6 million years ago.
Geological epoch dating from 2.6 million years ago to about 11,000 years ago.
Dating methods that give estimated numbers of years for artifacts and sites.
Matter that cannot be broken down into smaller matter.
A small building block of matter.
Variants of elements that do not change over time without outside interference.
Variants of elements that spontaneously change into stable isotopes over time.
The chronometric dating method based on the radioactive decay of 14C in organic remains.
A chronometric dating method that uses the annual growth of trees to build a timeline into the past.
Microscopic single-celled organisms with a shell that are common in all marine environments. The fossil record of foraminifera extends back well over 500 million years.
A chronometric dating method that measures the ratio of argon gas in volcanic rock to estimate time elapsed since the volcanic rock cooled and solidified. See also argon-argon dating.
A chronometric dating method that measures the ratio of argon gas in volcanic rock to estimate time elapsed since the volcanic rock cooled and solidified. See also potassium-argon dating.