3 The Code of Life
Learning Objectives
- Outline a 21st-century perspective of the Modern Synthesis.
- Define populations and population genetics as well as the methods used to study them.
- Identify the forces of evolution and become familiar with examples of each.
- Discuss the evolutionary significance of mutation, genetic drift, gene flow, and natural selection.
- Explain how allele frequencies can be used to study evolution as it happens.
- Contrast micro- and macroevolution.
My son is colorblind and has trouble distinguishing red and green and a few other colors. For a long time, he thought pumpkins were yellow and that we had a green couch. Now we refer to that couch as the “green couch” using finger quotes. His colorblindness is a result of a genetic mutation. In other cases, genetic mutations are more dramatic. On the island of Pingelap, about one in ten people are completely colorblind. That is, they see only in black and white. In the world’s population, the incidence of total colorblindness or achromatopsia is rare, only 1 in 40,000. How is it that so many people on Pingelap are totally colorblind then? One idea is that a catastrophic typhoon hit the island of Pingelap in 1780 and the king, who had achromatopsia, was one of only 20 people that survived. Given the limited number of people, and perhaps the king’s personal rizz, the mutation for the condition spread over time. Unfortunately, this form of colorblindness also makes people very sensitive to the sun, and they can’t see very well at all during the daytime. One solution has been to fish at night, at which the Pingelap people excel. The achromatopsia mutation is an example of a population bottleneck, when the size of a population drops precipitously. Bottlenecks in turn influence the future prevalence of traits in the general population of a group. This chapter explores human variation, how we are the same and how we are different, and how we got to look the way we do.
The Code of Life
In 2003, The Human Genome Project mapped out the entire human genome, the entire set of genetic instructions for our species. All humans are, genetically speaking, very much alike, sharing 99.9 percent of our DNA in common. However, people aren’t identical and genes differ from one person to another. Phenotype refers to the expression of genes, such as height or hair color. Some phenotypic variation is not outwardly obvious, like blood type. When we talk about the genes themselves we use the term genotype. So how and why do humans vary?
DNA (deoxyribonucleic acid) is composed of nucleotides, which are molecules made up of sugars, phosphates, and nucleotide bases (Figure 3.1). There are only four bases: adenine, thymine, cytosine, guanine, and thymine—A, T, C, and G for short. These bases are paired in a weak cchemical bond, A with G and C with T. Just as zeros and ones make up current computer systems, these four letters form the basis of all life on earth, from nematodes to narwhals. The bases are paired with one another—A with T and G with C—to form the steps of the DNA spiral ladder or double helix. The human genome, the complete set of genetic material, contains about 3 billion base pairs. Almost all the cells in your body, within the nucleus of each cell, contain all of your DNA or genetic instructions. Needless to say, your cells do an excellent job of packing the DNA and economizing space. If your cells were in charge of putting away the holiday lights (with only four colors, AGCT), they would be extremely efficient with no hopelessly tangled balls of wire. The system is so efficient in terms of space, if you took all the DNA in all your cells and strung it out end to end, you could make it out of the solar system! (Bryson 2019) But before we start celebrating our vast genome, it is now known that the axolotl, a kind of salamander native to Mexico, has 32 billion base pairs (Bakalar 2018). That’s 10 times the size of the human genome. This huge genome is likely related to the fact that axolotls can regrow limbs (Figure 3.2). So much for human exceptionalism in the base pair department.
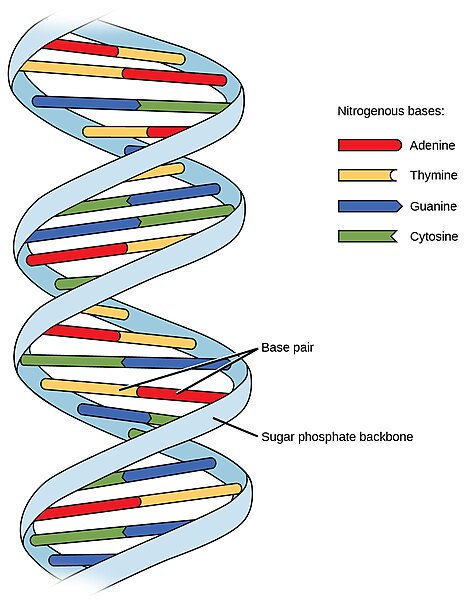
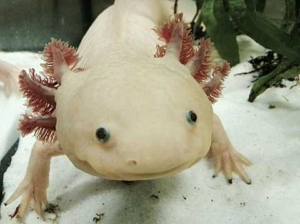
Some sequences of nucleotide bases in the genome code for proteins. We call these genes. It wasn’t so long ago that we did not know the total number of genes in the human genome. In the History of Everyone Who Ever Lived, geneticist Adam Rutherford describes how the world’s pre-eminent geneticists placed bets on the number of genes in the human genome, anticipating the results of the Human Genome Project. Some experts guessed around 100,000 genes. Today we know rice has 50,000 genes, bananas have 36,000, and the “water flea” 31,000. Humans, as revealed by the Human Genome Project, crossed the finish line at a disappointing 20,000 genes.
Exercises
In addition to genes that code for protein, there are non-coding regions as well. Formerly, these were thought to be junk, leftover genetic odds and ends that once had a purpose. However, more recent research has shown that these noncoding genomic regions influence how individual genes are turned “on” and “off” or regulated. These regulator genes could play a large role in phenotypic differences. These regions that are responsible for turning other genes on and off are often the focus of research into disease and gene therapy. Turning “off” the expression of a harmful gene variant could dampen or prevent its harmful effects (Mann, Explorations).
Mistakes Are Made: Mutations
Occasionally, when DNA replicates, a copying error or mutation occurs. Mutations are the original source of variation in all life. Without mutation, we would all be genetically identical and life would be pretty dull. Mutations occur randomly. With every child born, there are approximately 50 new mutations. A common type of mutation is a shift in a single letter (nucleotide base), in which one letter is substituted for another. These mutations are called SNPs (“snips”), short for single nucleotide polymorphisms. A polymorphism means there are multiple forms of a trait, like hair color or blood type. These mutations are also called point mutations. SNPs are the most common form of genetic difference between people. Sometimes a SNP has no effect. Other times, a SNP can affect a physical trait like eye color. And in rare cases, a SNP can lead to a debilitating disease like cystic fibrosis, sickle-cell anemia, or Huntington’s disease. These SNPs that cause harm are referred to as deleterious, which is a fabulous word for a not-so-fabulous thing.
Mutations occur regularly, but luckily our DNA has a repair system that swoops in and corrects the changes. One source of mutation in living cells in UV radiation in sunlight which can cause mutations in the skin. These mutations can lead to pigmented areas like freckles or moles as a protective response. The mutations can also potentially lead to skin cancer. This is why sunscreen is important.
SNPs, a change in a single letter (nucleotide base), account for much of human variation. Two people chosen at random are likely to differ in 1 in every 1,000 nucleotide bases or 0.1 percent. With the advent of technology that can identify certain SNPs from a sample of spit, we are learning more about how SNPs are distributed around the world and what their effect is if any. There’s even a website called “SNPedia” that provides information about different SNPs. Other types of changes in bases can happen as well, including inversion, insertion, deletion, or duplication of bases, which can result in human variation.
Exercises
Mutations can pop up spontaneously, which is aptly known as spontaneous mutation. A famous spontaneous mutation that caused disease (deleterious) originated with Queen Victoria of Great Britain (Figure 3.3). She carried a mutation for hemophilia, a blood clotting disease. The SNP is thought to have arisen through spontaneous mutation. While the disease is not expressed in females, it is expressed in males. Queen Victoria passed the mutation on to some of her daughters and sons. Her children and grandchildren married nobility from all over Europe and Russia, spreading the SNP to several royal families, earning the name “royal’s disease.”
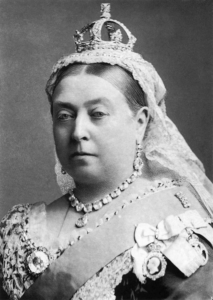
Errors can occur at other levels of the genome. Our DNA is packaged into units called chromosomes If the genes are Pokemon cards, then chromosomes are the binders where you store them. Humans have 23 pairs of chromosomes, hence the name of the popular genetic testing company 23andme. There are two sex chromosomes, X and Y. Genetic males have XY and genetic females have XX. However, it is possible to have the conditions XXX, XXY, XYY and X. In later chapters, we will see that these conditions have been detected in individuals from archaeological sites.
People inherit genes from both their mother and their father. The genes you inherit from each parent may be slightly different. These variants of genes, called alleles, are sometimes either dominant or recessive. A dominant allele will mask the expression of the recessive allele. Sometimes, you need two copies of the recessive allele for the disease to be expressed. Inbreeding within a population can lead to problems because a person is more likely to inherit two copies of a faulty allele. Inbreeding simply means that mate selection is occurring within a small population. The relatively limited variability can lead to the expression of deleterious genes. Carlos II (1661-1700), King of Spain, is a case in point (Figure 3.4). To protect the Spanish Hapsburg dynasty, marriages between cousins and between uncles and nieces were common in the royal line. As a result, Carlos’ family tree collapsed in on itself. Where Carlos should have been 64 ancestors, he had only 32 (Rutherford 2016). The result was that Carlos was physically and mentally infirm along with being infertile.
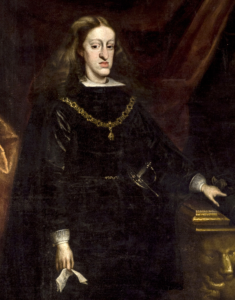
Genetic Testing
The following is from Chapter 3 “Molecular Biology and Genetics” of Explorations, An Open Invitation to Biological Anthropology (second edition) by Haley Mann. To assist with public health efforts, newborn screening for genetic diseases has been available in the United States for over 50 years. One of the first available genetic tests was to confirm a phenylketonuria (PKU) diagnosis in infants, which is easily treatable with a dietary change. Currently, each state decides what genes are included on newborn screening panels and some states even have programs to help with infant medical follow-ups.
There are now hundreds of laboratories that provide testing for a few thousand different genes that can inform medical decisions for infants and adults. What has made this industry possible are the advancements in technology and decreased cost to patients. Moreover, genetic testing has been made available publicly to anyone without the assistance of medical professionals.
Clinical Testing
Clinical genetics tests assist patients with making medically informed decisions about family planning and health. Applications of this technology include assistance with in vitro fertilization (IVF) procedures, embryo genetic screening, and personalized medicine such as matching patients to cancer therapies. To ensure accuracy of patient genetic screening, it is important that all clinical laboratories are regulated. The Clinical Laboratory Improvement Amendments (CLIA) are United States federal standards that all human laboratory testing clinics must follow. A major benefit provided by some clinical genetic testing companies is access to genetic counselors, who have specialized education and training in medical genetics and counseling. For individuals with a family history of genetic disease, a physician may recommend genetic carrier screening to see if there is a risk for passing on a disease to a child. Genetic counselors provide expertise with interpretation of genetic testing results, as well as help guide and support patients when making impactful medical decisions.
Direct-to-Consumer (DTC) Genetic Testing
Genetic testing that is performed without the guidance of medical professionals is called direct-to-consumer (DTC) genetic testing. Companies that sell affordable genome sequencing products to the public continue to increase in popularity. These companies have marketing campaigns typically based on the notion of personal empowerment, which can be achieved by knowing more about your DNA. For example, if you are identified as having a slightly increased risk for developing celiac disease (Figure 3.36), then you may be motivated to modify your dietary consumption by removing gluten from your diet. Another scenario is that you could test positive for a known pathogenic BRCA1 or BRCA2 cancer-predisposing allele. In this case, you may want to follow up with a physician and obtain additional clinical testing, which could lead to life-altering decisions. DNA sequencing products for entertainment and lifestyle purposes are also available. For example, some DTC companies offer customized genetic reports for health and fitness, wherein recommendations for optimal exercise workout and meal plans are provided.
DTC testing typically lacks genetic counselor services to consumers, and regulations for nonclinical laboratories are not as strict. This has led to some controversies regarding company genetic products that provide health information. The company 23andMe was the first on the market to offer DTC health testing, and in 2013, the U.S. Food and Drug Administration (FDA) intervened. 23andMe worked toward complying with FDA regulations and then gained approval to offer testing on a few medically related genes. In 2017, 23andMe offered a “Late-Onset Alzheimer’s Disease” genetic risk report. Such offerings have been criticized because customers could receive results they may not fully be able to interpret without professional assistance and advice. In turn, this could increase the stress of participants (sometimes called the “burden of knowing”) and could lead to unnecessary medical intervention.To address this issue, 23andMe now provides disclaimers and also interactive learning modules that customers must complete if they wish to view certain genotyping results. However, individuals who tested positive for a disease-causing allele have also been able to successfully seek medical help. The potential for harm and the proposed benefits of DTC testing continue to be a topic of debate and investigation.
Populations Change over Time
Why do populations look different from others? There are two important ways in which SNPs get distributed that we will discuss: founder effect and natural Selection. The founder effect happens when a small group of people establishes a population, bringing with them a limited about of genetic variation. The founding group is then isolated from others. Sometimes the founders bring with them chance mutations, so subsequent generations are more likely to have those mutations as well. For example, a form of dwarfism Ellis-van Creveld accompanied by polydactyly, having extra fingers, occurs in Amish populations in Pennsylvania due to the Founder Effect. Fleeing religious persecution, a small group of Amish, around 200, came to Pennsylvania in 1744 bringing the mutation along with them. The mutation can be traced back to a single couple (the “founders”) Samuel Kind and his wife. The Amish practiced endogamy (marriage within the group) and the recessive gene for the condition was, therefore, more likely to occur than if they practiced exogamy (marriage outside the group). Andrea J. Alveshere, “Chapter 4: Forces of Evolution” by Andrea J. Alveshere. In Explorations: An Open Invitation to Biological Anthropology, writes, “Among the world population, Ellis-van Creveld syndrome is estimated to affect approximately 1 in 60,000 individuals; among the Old Order Amish of Lancaster County, the rate is estimated to be as high as 1 in every 200 births (D’Asdia et al. 2013).”
Another case of Founder Effect comes from the remote island of Tristan da Cunha in the far south Atlantic. There are just 300 residents living on the island, but more than half of them suffer from asthma (Zamel et al. 1996). Why? Because of the 15 original settlers, two were asthma sufferers. Iceland’s founders are well documented through Íslendingabók, The Book of Icelanders. Many people are related but may not know it. Because of the problem of potentially inheriting two copies of a deleterious (bad) gene or accidentally dating a cousin, some enterprising Icelanders developed the Islendiga-App, which warns you about dating potential relatives. If you bump phones and an alarm goes off, you may want to look elsewhere.
Northern New Mexico has also been shaped by founder effect. Many people in northern New Mexico have inherited a mutation that causes cerebral cavernous malformation (CCM). The disease causes blood vessels to form clusters in the brain and spinal cord producing headaches, seizures, and potentially strokes. The disease is caused by a mutation in the CCM1 gene, and unfortunately, the gene is dominant, so a person who has the mutation has a 50 percent chance of passing it on to their children. Some people with the gene have no symptoms, and there can be a wide range of severity. The disease derives from Cristóbal Baca, an early Spanish colonist of New Mexico in 1600. Researchers at the University of New Mexico Hospital and the Baca Family Historical Project are starting an effort to better diagnose people. (See this site if you have CCM and want to participate in a study to try to determine why the disease differs in severity).
Exercises
Bottlenecks
Bottlenecks occur where there is a sudden decline in a population. The effect is the same though as Founder Effect; there is less genetic variation in the population than in the original. On the Micronesian atoll of Pingelap, about one in ten people are totally colorblind, seeing only black and white. How did this happen? It is thought that a typhoon wiped out most of the population in 1775. The king, who was thought to have carried the defective gene, survived and passed it on to his many descendants. The typhoon then initiated a population bottleneck causing a change in allele frequencies. Interestingly though, those affected have excellent night vision and have taken up night fishing.
Other bottlenecks can have serious implications for human health as Andrea J. Alveshere explains in “Chapter 4, Forces of Evolution” in An Open Invitation to Biological Anthropology. Antibiotics are medicines prescribed to treat bacterial infections. The typical prescription includes enough medicine for ten days. People often feel better much sooner than ten days and sometimes decide to quit taking the medicine ahead of schedule. This is often a big mistake. The antibiotics have quickly killed off a large percentage of the bacteria—enough to reduce the symptoms and make you feel much better. However, this has created a bacterial population bottleneck. There are usually a small number of bacteria that survive those early days. If you take the medicine as prescribed for the full ten days, it’s quite likely that there will be no bacterial survivors. If you quit early, though, the survivors—who were the members of the original population who were most resistant to the antibiotic—will begin to reproduce again. Soon the infection will be back, possibly worse than before, and now all of the bacteria are resistant to the antibiotic that you had been prescribed. Geneticist Jerry Coyne points out that when penicillin was first introduced in the 1940s it could kill and could wipe out every strain of Staphylococcus aureus in the world. Today, more than 95% percent of staph strains are resistant to penicillin. Today, we are in a very real arms race with organisms that are invisible to the naked eye. In “Chapter 4: Forces of Evolution” in Explorations: An Open Invitation to Biological Anthropology, first edition Andrea J. Alveshere describes the problem. Viruses, bacteria, agricultural pests, and weeds have all shown great flexibility in developing alleles that make them resistant to the latest medical treatment, pesticide, or herbicide. Billion-dollar industries have specialized in trying to keep our species one step ahead of the next mutation in the pests and infectious diseases that put our survival at risk.
Exercises
Natural Selection
In natural selection, a trait has some advantage in a particular environment that causes individuals with that trait to survive and reproduce at a higher rate than individuals without the trait. Unlike founder effect, traits confer some advantage (or disadvantage) to the organism. A clear example of natural selection comes from the New Mexico rock pocket mouse. Mice that live in sandy desert areas of New Mexico are a sandy brown color, blending in with their environment from predators. But New Mexico also has several areas of lava flows like The Valley of Fire. In this region, mice tend to be grayer, blending in with the lava flow. More sandy coloration is a liability on the darker lava, and those mice are selected out of the population by being eaten by predators leaving the darker mice to survive and reproduce at a greater rate. Genetic studies show mutations in the MC1R gene leading to dark coloration. On different lava flows different mutations have been selected for resulting in dark coloration. Even if the dark mice have just a 1 percent advantage, 95% percent of mice will be dark in 1,000 years (HHMI). Importantly, the mutations occur at random, but natural selection acts upon those random mutations. This is called directional selection because the color of the mouse is going toward an extreme, dark on volcanic flows and light on sand.
The same process, natural selection, that has shaped the rock pocket mouse has shaped the human phenotype, from lactose tolerance (a.k.a. Lactase persistence) to disease resistance to skin color. Human babies can digest lactose until about the age of 4 to 6, then the ability shuts down and people become lactose intolerant. In this case, the gene does not code for a protein but switches on and off the gene that produces lactase. In some populations, SNPs arose such that the ability to digest lactose never switches off and adults can drink milk freely. Not too surprisingly, populations that can drink milk are those where herding animals are important, or were important in the past, to their ancestral populations. This is a good example of how a cultural trait, herding, led to the selection of a biological trait, lactose tolerance. In fact, humans are the only mammal that can do this. Shaping our biology as a result of culture is called gene-culture co-evolution. The ability to digest milk, even if it conferred a small advantage, would have led to most people being lactose tolerant, similar to the pocket mouse example.
As with the pocket mouse example, different mutations led to lactose tolerance in different populations. Different SNPs account for lactose tolerance in Europe, Africa, and the Middle East. The same SNP accounts for lactose tolerance in Europe and India, indicating a common origin. In northern Europeans, lactose tolerance is a result of just one SNP, a mutation in a single base pair. The Masai cattle herders of Tanzania and other sub-Saharan Africans, however, have a different mutation that also results in lactose tolerance (Figure 3.5). Like the pocket mice, these mutations popped up randomly but were acted on through natural selection because they were advantageous in cultures with domesticated animals that could be milked.
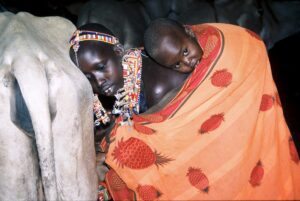
Skin Color
All primates can produce melanin in their skin, which is a natural sunscreen. Skin color depends on the type of melanin produced in skin cells called melanocytes. Melanin also affects what eye color you have. Blue eyes derive from a mutation in a genetic “switch” for the OCA2 gene (Eiberg 2008). The switch reduces the ability of the gene to produce melanin in the eye, resulting in blue eyes. Melanin also colors animal hair and bird feathers. But why does human skin color differ?
Ultraviolet radiation damages DNA in the skin and disrupts cell processes. Dark skin protects against ultraviolet radiation and the mutations it can cause. Melanin acts as a barrier between the ultraviolet rays and the nucleus of the skin cell, which houses the DNA. In short, melanin protects DNA and helps prevent skin cancers. Those with dark skin in high UV regions, which correlates generally with latitude, would have a selective advantage over lighter-skinned people. But protecting DNA in skin cells is not the only advantage. Dark skin also conserves folate, which is destroyed by ultraviolet-B sunlight (UVB). Folate is essential because low folate in pregnant women can result in severe birth defects called neural tube defects. After all, the demand for folate increases during pregnancy. Thus, dark skin confers a great advantage over light skin in the tropics, not just because it prevents skin cancers but also because it prevents birth defects. These advantages would quickly make dark skin universal in areas of high UV, much like the dark fur on rock pocket mice on lava flows. Because folate is destroyed by sunlight, pregnant women take folic acid or eat foods high in folate to prevent neural tube defects. In the United States, many foods like cereals, bread, and pasta are supplemented with folic acid for this reason.
We can then ask, why do some people have lighter skin? Lighter skin is a liability in the tropics, but those with lighter skin can better synthesize vitamin D (a hormone necessary for the absorption of calcium) in more northerly climates. Melanin greatly slows the production of vitamin D. A very dark-skinned person will take six times as long to produce the same amount of vitamin D as a light-skinned person. Nutritional rickets, a deforming bone disease, can develop in the presence of vitamin D deficiency. Women with rickets have reduced pelvic openings and have difficulty delivering babies, making the selective factors for light skin strong in some areas. Some of the first c-sections in the U.S. were on enslaved African American women who suffered from pelvic deformities, likely brought about by vitamin D deficiencies. Foods are now enriched with vitamin D to prevent Vitamin D deficiencies (in the past, kids took cod liver oil). Vitamin D deficiencies are now being recognized as having other effects, including a weakened immune system and an association with cancers. Like dark skin in the tropics, light skin is naturally selected for in areas of lower ultraviolet radiation.
Exercises
There are still other skin phenotypes that are the product of natural selection. The ability to tan is also an adaptive trait based on genetic and environmental interaction. Tanning is the ability to increase melanin production when needed. In some areas of the world, the amount of sunlight varies considerably from one season to the next (e.g., the Mediterranean). Local populations have physiological mechanisms to darken their skin during yearly seasons of high sunlight. In other areas of the world, cloudy conditions prevail even during summer seasons, and very little tanning ability has evolved (British Isles).
Special Topic: Sickle Cell Anemia
Sometimes deleterious alleles occur at higher rates than would be expected. How can this be if natural selection is at work? Andrea J. Alveshere explains in Chapter 4: Forces of Evolution. Sickle cell anemia is an autosomal recessive genetic disorder that affects millions of people worldwide. It is most common in Africa, countries around the Mediterranean Sea, and eastward as far as India. Populations in the Americas that have high percentages of ancestors from these regions also have high rates of sickle cell anemia. In the United States, it’s estimated that 72,000 people live with the disease, with one in approximately 1,200 Hispanic-American babies and one in every 500 African-American babies inheriting the condition (World Health Organization 1996).

Figure 3.6: Sickle cell anemia. Arrows indicate (a) sickled and (b) normal red blood cells. Credit: Sickle-cell smear 2015-09-10 by Paulo Henrique Orlandi Mourao has been modified (contrast modified and labels added) and is under a CC BY-NC-SA 4.0 License.
Sickle cell anemia affects the hemoglobin protein in red blood cells. Normal red blood cells are somewhat doughnut-shaped—round with a depression on both sides of the middle. They carry oxygen around the bloodstream to cells throughout the body. Red blood cells produced by the mutated form of the gene take on a stiff, sickle-like crescent shape when stressed by low oxygen or dehydration (Figure 3.6). Because of their elongated shape and the fact that they are stiff rather than flexible, they tend to form clumps in the blood vessels, inhibiting blood flow to adjacent areas of the body. This causes episodes of extreme pain and can cause serious problems in the oxygen-deprived tissues. The sickle cells also break down much more quickly than normal cells, often lasting only 20 days rather than the 120 days of normal cells. This causes an overall shortage of blood cells in the sickle cell patient, resulting in low iron (anemia) and problems associated with it such as extreme fatigue, shortness of breath, and hindrances to children’s growth and development.
The devastating effects of sickle cell anemia made its high frequency a pressing mystery. Why would an allele that is so deleterious in its homozygous form be maintained in a population at levels as high as the one in twelve African Americans estimated to carry at least one copy of the allele? The answer turned out to be one of the most interesting cases of balancing selection in the history of genetic study.
While looking for an explanation, scientists noticed that the countries with high rates of sickle cell disease also shared a high risk for another disease called malaria, which is caused by infection of the blood by a Plasmodium parasite. These parasites are carried by mosquitoes and enter the human bloodstream via a mosquito bite. Once infected, the person will experience flu-like symptoms that, if untreated, can often lead to death. Researchers discovered that many people living in these regions seemed to have a natural resistance to malaria. Further study revealed that people who carry the sickle cell allele are far less likely to experience a severe case of malaria. This would not be enough of a benefit to make the allele advantageous for the sickle cell homozygotes, who face shortened life spans due to sickle cell anemia. The real benefit of the sickle cell allele goes to the heterozygotes.
People who are heterozygous for sickle cell carry one normal allele, which produces the normal, round, red blood cells, and one sickle cell allele, which produces the sickle-shaped red blood cells. Thus, they have both the sickle and round blood cell types in their bloodstream. They produce enough of the round red blood cells to avoid the symptoms of sickle cell anemia, but they have enough sickle cells to provide protection from malaria.
When the Plasmodium parasites infect an individual, they begin to multiply in the liver, but then must infect the red blood cells to complete their reproductive cycle. When the parasites enter sickle-type cells, the cells respond by taking on the sickle shape. This prevents the parasite from circulating through the bloodstream and completing its life cycle, greatly inhibiting the severity of the infection in the sickle cell heterozygotes compared to non–sickle cell homozygotes.
Biology and Destiny
All traits are genetic. Even if you spend hours at the gym and are ripped, that is still a genetic trait. How can that be? Genes produce chemicals that respond to all that iron pumping, ultimately creating big muscles. But all traits are also environmental. Wait, what? That’s right, the old nature-nurture debate has long been put to rest. For example, height has a genetic component, but diet also plays a role in how tall you will be. This is why people have become taller over the generations. Better nutrition and simply more food allow genes to reach their height potential. Superman is a good analogy. Under a red sun, he’s just your ordinary, mild-mannered, Clark Kent type. Under a yellow sun, he can fly and has x-ray vision. A yellow sun permits Superman’s genes to achieve their potential. This happens in other species as well. Himalayan rabbits raised in cold temperatures will have dark pigments on their nose, paws, and tail, and those raised at warmer temperatures are all white (Lobo, 2008). In this case, temperature affects whether a gene will be expressed or not. The temperature at which alligators are incubated determines whether they will be born male or female. Grasshoppers born into crowded conditions will have increased hopping ability, presumably to more easily migrate out of their current habitat. Genes and environment can’t be separated; instead, they interact to produce traits. Some traits, however, are more heritable than others, which means that the environment plays a lesser role in their expression. Rather than “nature versus nurture”, it is more accurate to speak of “nature through nurture.”
Exercises
Sometimes the media portrays traits as purely genetic. For example, a non-mutated gene ACTN3 occurs in virtually all Olympic power athletes like sprinters and power-lifters (MacArthur 2008). The gene variant produces a protein that allows for fast muscle contraction. One company called ATLAS at one point even marketed to parents to identify children’s version of the gene, to direct their kids into the correct sport. But the gene, of course, isn’t near the whole story. First, like other complex traits, other genes also influence athletic ability (though they are still poorly understood). And environmental factors, namely diet, and high-level training, also affect athletic success. What if you have the fast-twitch gene, but sit in a cubicle all day? Will you still become an Olympic athlete? Not likely.
Jamaicans have high frequencies of the ACTN3 gene and have dominated sprinting in the Olympics. But, running is a national pastime in Jamaica, comparable to football in the United States. In fact, the gene is thought to predict just 2–3 percent of the muscle variation in the general population. To illustrate this point, Usain Bolt very likely has at least one fast-twitch allele for ACTN3, but so does your anthropology instructor (Figure 3.7). The presence of the variants of ACTN3 can’t be the whole story. Genes and environment work together. Studies vary widely, finding that ACTN3 only accounts for 0 to around 2 percent difference in sprinting speed (Lieberman 2021). In his book Exercised, Lieberman suggests that there are likely many genes that influence athletic ability, just as there are more than 400 genes that influence height. There is no single gene that accounts for running ability within populations or between populations (Lieberman 2021).
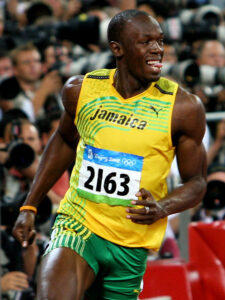
Race
Racial classification involves assigning humans to categories based on phenotype, especially traits that are outwardly apparent. In the recent past, American children were taught that there are 5 great races of the world, a taxonomy based on Johann Blumenbach’s racial hierarchy of the late 1790s. This is the essentialist view of race—that there are discrete groups with large gaps between them. According to Blumenbach, “Caucasians” were the most ideal form, and everyone was of a degenerate form of human.
The essentialist view of race runs into immediate problems in light of the continuous nature of traits, especially skin, hair, and eye color. Skin color and eye color are not straightforward SNPs like lactose tolerance or sickle cell trait. Rather, they are influenced by several different genes, more than 15 for skin and 16 or more for eye color. As a result, skin color can be “blended” in children of parents with different levels of skin pigmentation. Then, skin color is not an essentialist category, but rather a continuum. If skin color is meant to reflect deeper qualities of a person or a person’s wider genotype, what do we make of siblings, even fraternal twins, who are considered different races based on their skin color? And how does a single individual who shares perceived features of different races—like dark skin and blonde hair— get categorized? For instance, blonde hair arose independently in Europe and Melanesia (Figure 3.8). (In Melanesia the blonde trait is caused by a single SNP, a C switched to a T). How would these people be categorized based on phenotype? Which trait is considered more important to race, and why? People have to decide, culturally, whether someone belongs to a race or not.
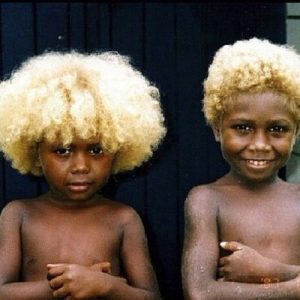
With the Human Genome Project results, we know more about the genotypes of human populations. For instance, we now know that the genetic variation between any two individuals is about .1 percent. Since we have 3 billion pairs of bases, that means about 3 million differences. Most genetic variation, SNPs, and other forms of mutation (85–95%) are found within populations—nations and linguistic groups— around the world. As writer Malik Kenan in “Why Both Sides are Wrong in the Race Debate” writes, “Imagine that some nuclear nightmare wiped out the entire human race apart from one small population—say, the Masai tribe in East Africa. Almost all the genetic variation that exists in the world today would still be present in that one small group.” A smaller percentage of genetic variation occurs between supposed races, about 3–5 percent. Maybe this 3 to 5 percent difference is useful for dividing up people? As Kenan points out, the differences between human populations are purely statistical, not essential or absolute. This means populations are more likely to contain certain alleles, but not everyone has them. Secondly, statistical differences could be found between virtually any two populations, such that there could theoretically be hundreds or thousands of races based on genetics if we decided that those categories would be useful and productive for society or science. If you’re looking for statistical genetic differences between populations, you will find them. Even if there are genetic differences between populations, complex traits like athleticism, intelligence, musicality, and so forth are not influenced by a few genes, but likely hundreds of genes as well as the environment. We always have to decide which criteria, whether phenotypic or genotypic, to base our categories, and also we need to consider why these categories are needed.
In another light, race is very much real even though as a biological category it has little value. Culturally, race is very much real. We know that we humans create our world—we decide that money, companies, borders, and so forth—are real things. The same is true for race. The great lengths to which people will go to create racial categories are enlightening. In the United States, for example, the one drop rule meant that any African ancestry qualified someone as being black, but not white, even if most of their ancestors were European and light-skinned. As a result, Barack Obama was the first black president of the United States, even though his anthropologist mother was considered white. Some racial categories are ridiculously specific. For example, Louisiana had several categories for people of African descent, including quadroon (¼ African), octoroon (⅛ African), and even hexadecaroon (1/16 African). In other cases, racial categories were explicitly cultural. Pilar Ossorio, legal scholar, microbiologist, and bioethicist writes:
“And historically, in order to be a naturalized citizen in this country, as an immigrant, you had to be categorized as white or black. So the courts had to make decisions about who was white and who was not. Is an Armenian person white? There were several cases dealing with Asian people, and are they white or not white. And so one of the things that would happen is the person would come into court and say, “Look, my skin color is as white as anybody else’s skin color in here who is categorized as white. The court often decided who was white and who wasn’t based on whether they felt that the person would politically fit well into the kind of society we were trying to build.”
Individuals have gone to great lengths to be recognized as a particular race. A 1970 law in Louisiana stated that 1/32 African-American genes qualified an individual as black. Susie Guillory Phipps, who considered herself white, discovered she was legally considered African American when she ordered a copy of her birth certificate. The Phipps family began a 20,000-dollar legal battle to get the law declared unconstitutional and to get Susie Phipps officially declared white.
Kim Tallbear (2014) explains how racial classification has affected tribal membership for native people: “In most US tribes, you have a specific blood quantum needed for enrollment – often one-quarter. That means you have to be able to show with paper documentation that you have one out of four grandparents who is full blood. Or you might have two grandparents who are half-blood – however, you can make those fractions work.” According to TallBear, some tribes have turned to DNA testing to determine who qualifies for membership. While genetic testing can determine paternity, no test can determine a genetic link to a particular tribe. Native identity is not racial, but rather, Tallbear argues, being culturally part of an indigenous community. Relying on biology as a qualification for tribal membership, she argues, means handing over control of tribal identity to genetic research institutions (Tallbear 2016).
Exercises
Race and Forensic Anthropology
Forensic anthropologists examine human remains to try to identify a deceased individual to be used as evidence in court. To achieve this, forensic anthropologists examine bones and sometimes DNA to estimate the individual’s stature, health, injuries, sex, age, how they lived, and outward appearance. Forensic anthropologists will also use cultural cues, like clothing, jewelry, and other effects, to aid in the identification. Work is often conducted with unidentified soldiers’ remains, mass graves, victims of terrorism, natural disaster victims, crime victims, and sometimes bodies in unmarked graves.
We know that race is not an essentialist category with all members of a perceived race having all genetic variants. Andrea J. Alveshere explains, “Among humans, gene flow is often described as admixture. In forensic cases, anthropologists and geneticists are often asked to estimate the ancestry of unidentified human remains to help determine whether they match any missing persons’ reports. This is one of the most complicated tasks in these professions because, while “race” or “ancestry” involves simple checkboxes on a missing person’s form, among humans today there are no truly distinct genetic populations. All modern humans are members of the same fully breeding compatible species, and all human communities have experienced multiple episodes of gene flow (admixture), leading all humans today to be so genetically similar that we are all members of the same (and only surviving) human subspecies: Homo sapiens sapiens.”
Key Terms
5-alpha reductase deficiency: An autosomal recessive syndrome that manifests when a child having both X and Y sex chromosomes inherits two nonfunctional (mutated) copies of the SRD5A2 gene, producing a deficiency in a hormone necessary for development in infancy of typical male genitalia. These children often appear at birth to have female genitalia, but they develop a penis and other sexual characteristics when other hormones kick in during puberty.
Adaptive radiation: The situation in which subgroups of a single species rapidly diversify and adapt to fill a variety of ecological niches.
Admixture: A term often used to describe gene flow between human populations. Sometimes also used to describe gene flow between nonhuman populations.
Allele frequency: The ratio, or percentage, of one allele compared to the other alleles for that gene within the study population.
Alleles: Variant forms of genes.
Allopatric speciation: Speciation caused by long-term isolation (physical separation) of subgroups of the population.
Antibiotics: Medicines prescribed to treat bacterial infections.
Artificial selection: Human-directed assortative mating among domestic animals, such as pets and livestock, designed to increase the chances of offspring having certain desirable traits.
Asexual reproduction: Reproduction via mitosis, whereby offspring are clones of the parents.
Autosomal dominant: A phenotype produced by a gene on an autosomal chromosome that is expressed, to the exclusion of the recessive phenotype, in heterozygotes.
Autosomal recessive: A phenotype produced by a gene on an autosomal chromosome that is expressed only in individuals homozygous for the recessive allele.
Balanced translocations: Chromosomal translocations in which the genes are swapped but no genetic information is lost.
Balancing selection: A pattern of natural selection that occurs when the extremes of a trait are selected against, favoring the intermediate phenotype (a.k.a. stabilizing selection).
Beneficial mutations: Mutations that produce some sort of an advantage to the individual.
Benign: Noncancerous. Benign tumors may cause problems due to the area in which they are located (e.g., they might put pressure on a nerve or brain area), but they will not release cells that aggressively spread to other areas of the body.
Café-au-lait spots (CALS): Flat, brown birthmark-like spots on the skin, commonly associated with Neurofibromatosis Type 1.
Chromosomal translocations: The transfer of DNA between nonhomologous chromosomes.
Chromosomes: Molecules that carry collections of genes.
Codons: Three-nucleotide units of DNA that function as three-letter “words,” encoding instructions for the addition of one amino acid to a protein or indicating that the protein is complete.
Cretaceous–Paleogene extinction: A mass disaster caused by an asteroid that struck the earth approximately 66 million years ago and killed 75% of life on Earth, including all terrestrial dinosaurs. (a.k.a. K-Pg Extinction, Cretatious-Tertiary Extinction, and K-T Extinction).
Crossover events: Chromosomal alterations that occur when DNA is swapped between homologous chromosomes while they are paired up during meiosis I.
Cutaneous neurofibromas: Neurofibromas that manifest as spherical bumps on or just under the surface of the skin.
Deleterious mutation: A mutation producing negative effects to the individual such as the beginnings of cancers or heritable disorders.
Deletions: Mutations that involve the removal of one or more nucleotides from a DNA sequence.
Derivative chromosomes: New chromosomal structures resulting from translocations.
Dictyostelium discoideum: A species of social amoebae that has been widely used for laboratory research. Laboratory strains of Dictyostelium discoideum all carry mutations in the NF1 gene, which is what allows them to survive on liquid media (agar) in Petri dishes.
Directional selection: A pattern of natural selection in which one phenotype is favored over the other, causing the frequencies of the associated advantageous alleles to gradually increase.
Disruptive selection: A pattern of natural selection that occurs when both extremes of a trait are advantageous and intermediate phenotypes are selected against (a.k.a. diversifying selection).
DNA repair mechanisms: Enzymes that patrol and repair DNA in living cells.
DNA transposons: Transposons that are clipped out of the DNA sequence itself and inserted elsewhere in the genome.
Ecological niche: A set of constraints and resources that are available in an environmental setting.
Ellis-van Creveld syndrome: An autosomal recessive disorder characterized by short stature (dwarfism), polydactyly (the development of more than five digits [fingers or toes] on the hands or feet), abnormal tooth development, and heart defects. Estimated to affect approximately one in 60,000 individuals worldwide, among the Old Order Amish of Lancaster County, the rate is estimated to be as high as one in every 200 births.
Evolution: A change in the allele frequencies in a population over time.
Exons: The DNA sequences within a gene that directly encode protein sequences. After being transcribed into messenger RNA, the introns (DNA sequences within a gene that do not directly encode protein sequences) are clipped out, and the exons are pasted together prior to translation.
Fertile offspring: Offspring that can successfully reproduce, resulting in offspring of their own.
Founder effect: A type of genetic drift that occurs when members of a population leave the main or “parent” group and form a new population that no longer interbreeds with the other members of the original group.
Frameshift mutations: Types of indels that involve the insertion or deletion of any number of nucleotides that is not a multiple of three. These “shift the reading frame” and cause all codons beyond the mutation to be misread.
Gametes: The reproductive cells, produced through meiosis (a.k.a. germ cells or sperm or egg cells).
Gene: A sequence of DNA that provides coding information for the construction of proteins.
Gene flow: The movement of alleles from one population to another. This is one of the forces of evolution.
Gene pool: The entire collection of genetic material in a breeding community that can be passed on from one generation to the next.
Genetic drift: Random changes in allele frequencies within a population from one generation to the next. This is one of the forces of evolution.
Genotype: The set of alleles that an individual has for a given gene.
Genotype frequencies: The ratios or percentages of the different homozygous and heterozygous genotypes in the population.
Guevedoces: The term coined locally in the Dominican Republic for the condition scientifically known as 5-alpha reductase deficiency. The literal translation is “penis at twelve.”
Hardy-Weinberg Equilibrium: A mathematical formula (1=p2 + 2pq + q2 ) that allows estimation of the number and distribution of dominant and recessive alleles in a population.
Harlequin ladybeetle: A species of ladybeetle, native to East Asia, that was introduced to Europe and the Americas as a form of pest control. After many decades of use, one of the North American strains developed the ability to reproduce in diverse environments, causing it to spread rapidly throughout the Americas, Europe, and Africa. It has hybridized with European strains and is now a major pest in its own right.
Heterozygous genotype: A genotype comprising two different alleles.
Homozygous genotype: A genotype comprising an identical set of alleles.
Hybridization: A term often used to describe gene flow between nonhuman populations.
Inbreeding: The selection of mates exclusively from within a small, closed population.
Indels: A class of mutations that includes both insertions and deletions.
Inherited mutation: A mutation that has been passed from parent to offspring.
Insertions: Mutations that involve the addition of one or more nucleotides into a DNA sequence.
Isolation: Prevention of a population subgroup from breeding with other members of the same species due to a physical barrier or, in humans, a cultural rule.
Last Universal Common Ancestor (LUCA): The ancient organism from which all living things on Earth are descended.
Macroevolution: Changes that result in the emergence of new species, how the similarities and differences between species, as well as the phylogenetic relationships with other taxa, lead to changes that result in the emergence of new species.
Malaria: A frequently deadly mosquito-borne disease caused by infection of the blood by a Plasmodium parasite.
Malignant: Cancerous. Malignant tumors grow aggressively and their cells may metastasize (travel through the blood or lymph systems) to form new, aggressive tumors in other areas of the body.
Microevolution: Changes in allele frequencies within breeding populations—that is, within a single species.
Modern Synthesis: The integration of Darwin’s, Mendel’s, and subsequent research into a unified theory of evolution.
Monosomies: Conditions resulting from a nondisjunction event, in which a cell ends up with only one copy of a chromosome. In humans, a single X chromosome is the only survivable monosomy.
Mutation: A change in the nucleotide sequence of the genetic code. This is one of the forces of evolution.
Natural selection: An evolutionary process that occurs when certain phenotypes confer an advantage or disadvantage in survival and/or reproductive success. This is one of the forces of evolution, and it was first identified by Charles Darwin.
Negative assortative mating: A pattern that occurs when individuals tend to select mates with qualities different from their own.
Neurofibromas: Nerve sheath tumors that are common symptoms of Neurofibromatosis Type 1.
Neurofibromatosis Type 1: An autosomal dominant genetic disorder affecting one in every 3,000 people. It is caused by mutation of the NF1 gene on Chromosome 17, resulting in a defective neurofibromin protein. The disorder is characterized by neurofibromas, café-au-lait spots, and a host of other potential symptoms.
NF1: An abbreviation for Neurofibromatosis Type 1. When italicized, NF1 refers to the gene on Chromosome 17 that encodes the neurofibromin protein.
Nondisjunction events: Chromosomal abnormalities that occur when the homologous chromosomes (in meiosis I) or sister chromatids (in meiosis II and mitosis) fail to separate after pairing. The result is that both chromosomes or chromatids end up in the same daughter cell, leaving the other daughter cell without any copy of that chromosome.
Nonrandom mating: A scenario in which mate choice within a population follows a nonrandom pattern (a.k.a. assortative mating).
Nonsynonymous mutation: A point mutation that causes a change in the resulting protein.
Old Order Amish: A culturally isolated population in Lancaster County, Pennsylvania, that has approximately 50,000 current members, all of whom can trace their ancestry back to a group of approximately eighty individuals. This group has high rates of certain genetics disorders, including Ellis-van Creveld syndrome.
Origins of life: How the first living organism came into being.
Peacock: The male sex of the peafowl, famous for its large, colorful tail, which it dramatically displays to attract mates. (The female of the species is known as a peahen.)
Peppered moth: A species of moth (Biston betularia) found in England that has light and dark phenotypes. During the Industrial Revolution, when soot blackened the trees, the frequency of the previously rare dark phenotype dramatically increased, as lighter-colored moths were easier for birds to spot against the sooty trees. After environmental regulations eliminated the soot, the lighter-colored phenotype gradually became most common again.
Phenotype: The observable traits that are produced by a genotype.
Phylogenetic tree of life: A family tree of all living organisms, based on genetic relationships.
Phylogenies: Genetically determined family lineages.
Plasmodium: A genus of mosquito-borne parasite. Several Plasmodium species cause malaria when introduced to the human bloodstream via a mosquito bite.
Plexiform neurofibromas: Neurofibromas that involve whole branches of nerves, often giving the appearance that the surface of the skin is “melting.”
Point mutation: A single-letter (single-nucleotide) change in the genetic code, resulting in the substitution of one nucleic acid base for a different one.
Polymorphisms: Multiple forms of a trait; alternative phenotypes within a given species.
Population: A group of individuals who are genetically similar enough and geographically near enough to one another that they can breed and produce new generations of individuals.
Population bottleneck: A type of genetic drift that occurs when the number of individuals in a population drops dramatically due to some random event.
Positive assortative mating: A pattern that results from a tendency for individuals to mate with others who share similar phenotypes.
Retrotransposons: Transposons that are transcribed from DNA into RNA, and then are “reverse transcribed,” to insert the copied sequence into a new location in the DNA.
Scutellata honey bees: A strain of honey bees that resulted from the hybridization of African and European honey bee subspecies. These bees were accidentally released into the wild in 1957 in Brazil and have since spread throughout South and Central America and into the United States. Also known as “killer bees,” they tend to be very aggressive in defense of their hives and have caused many fatal injuries to humans and livestock.
Sexual reproduction: Reproduction via meiosis and combination of gametes. Offspring inherit genetic material from both parents.
Sexual selection: An aspect of natural selection in which the selective pressure specifically affects reproductive success (the ability to successfully breed and raise offspring).
Sickle cell anemia: An autosomal recessive genetic disorder that affects millions of people worldwide. It is most common in Africa, countries around the Mediterranean Sea, and eastward as far as India. Homozygotes for the recessive allele develop the disorder, which produce misshapen red blood cells that cause iron deficiency, painful episodes of oxygen-deprivation in localized tissues, and a host of other symptoms. In heterozygotes, though, the sickle cell allele confers a greater resistance to malaria.
Somatic cells: The cells of our organs and other body tissues (all cells except gametes) that replicate by mitosis.
Speciation: The process by which a single population divides into two or more separate species.
Species: Organisms whose individuals are capable of breeding because they are biologically and behaviorally compatible to produce viable, fertile offspring.
Spontaneous mutation: A mutation that occurs due to random chance or unintentional exposure to mutagens. In families, a spontaneous mutation is the first case, as opposed to mutations that are inherited from parents.
Subspecies: A distinct subtype of a species. Most often, this is a geographically isolated population with unique phenotypes; however, it remains biologically and behaviorally capable of interbreeding with other populations of the same species.
Sympatric speciation: When a population splits into two or more separate species while remaining located together without a physical (or cultural) barrier.
Synonymous mutation: A point mutation that does not change the resulting protein.
Transposable elements: Fragments of DNA that can “jump” around in the genome.
Transposon: Another term for “transposable element.”
Trisomies: Conditions in which three copies of the same chromosome end up in a cell, resulting from a nondisjunction event. Down syndrome, Edwards syndrome, and Patau syndrome are trisomies.
Unbalanced translocations: Chromosomal translocations in which there is an unequal exchange of genetic material, resulting in duplication or loss of genes.
UV crosslinking: A type of mutation in which adjacent thymine bases bind to one another in the presence of UV light.
Viable offspring: Offspring that are healthy enough to survive to adulthood.
Attribution
This chapter is a mixture of the following sources.
Andrea J. Alveshere. “Chapter 4: Forces of Evolution,” In Explorations: An Open Invitation to Biological Anthropology, second edition, edited by Beth Shook, Lara Braff, Katie Nelson, and Kelsie Aguilera, which is licensed under CC BY-NC 4.0. (The second edition chapter is a revision of “Chapter 4: Forces of Evolution” by Andrea J. Alveshere. In Explorations: An Open Invitation to Biological Anthropology, first edition, edited by Beth Shook, Katie Nelson, Kelsie Aguilera, and Lara Braff, which is licensed under CC BY-NC 4.0.)
Hayley Mann, “Chapter 3: Molecular Biology and Genetics” In Explorations: An Open Invitation to Biological Anthropology (second edition), edited by edited by Beth Shook, Lara Braff, Katie Nelson, and Kelsie Aguilera, which is licensed under CC BY-NC 4.0. (The second edition chapter is a revision of “Chapter 3: Molecular Biology and Genetics” by Hayley Mann, Xazmin Lowman, and Malaina Gaddis. In Explorations: An Open Invitation to Biological Anthropology, first edition, edited by Beth Shook, Katie Nelson, Kelsie Aguilera, and Lara Braff, which is licensed under CC BY-NC 4.0.)
Susan Ruth, “Chapter 8: Human Variation,” In Being Human, which is licensed under CC BY-NC-SA 4.0.
Multiple forms of a trait; alternative phenotypes within a given species.
A mutation producing negative effects to the individual such as the beginnings of cancers or heritable disorders.
A mutation that occurs due to random chance or unintentional exposure to mutagens. In families, a spontaneous mutation is the first case, as opposed to mutations that are inherited from parents.
Molecules that carry collections of genes.
Variant forms of genes.
Medicines prescribed to treat bacterial infections.
A pattern of natural selection in which one phenotype is favored over the other, causing the frequencies of the associated advantageous alleles to gradually increase.
An autosomal recessive genetic disorder that affects millions of people worldwide. It is most common in Africa, countries around the Mediterranean Sea, and eastward as far as India. Homozygotes for the recessive allele develop the disorder, which produce misshapen red blood cells that cause iron deficiency, painful episodes of oxygen-deprivation in localized tissues, and a host of other symptoms. In heterozygotes, though, the sickle cell allele confers a greater resistance to malaria.
A frequently deadly mosquito-borne disease caused by infection of the blood by a Plasmodium parasite.
A genus of mosquito-borne parasite. Several Plasmodium species cause malaria when introduced to the human bloodstream via a mosquito bite.
A term often used to describe gene flow between human populations. Sometimes also used to describe gene flow between nonhuman populations.